At the Montanuniversität Leoben we have a new master program: The Erasmus Mundus Joint Master in Sustainable Mineral and Metal Processing Engineering-EMJM PROMISE. The program has been established because we see increasing demands in the quantity and diversity of minerals, metals, and materials as we move towards renewable energy, electromobility, digital communication and other clean-energy technologies.
PROMISE is the consortium involving the cooperation between four leading universities in mineral processing and mining engineering. Aside from our own university, the Montanuniversität Leoben in Austria (MUL), the University of Oulu from Finland (UOULU), University of Zagreb from Croatia (UNIZG), and Universidad Federico Santa Maria from Chile (USM) are partners.
Within the study programme 20 students moved in the Summer Semester 2023 to Leoben to complete their second semester. Besides the lecture, there is one main in the second semester, which is the composing of a project study related to mineral processing.
In this project study the connection between ReSoURCE and PROMISE has shown up. The first week of the semester is the so-called “Welcome Week” in which the project ReSoURCE was introduced to the students at the “Scientific Exchange Day” by myself. The idea of reducing the carbon footprint of RHI Magnesita with building up one of the first automated recycling plants for refractories brought a wide attention to the audience. Furthermore, a detailed introduction into the topic of electrodynamic fragmentation as alternate comminution technology was given.
Within the following weeks the students could gain knowledge to the processing of minerals in lectures and practical work in the technical facility of the Chair of Mineral Processing.
The ReSoURCE topic “The Use of Electrodynamic fragmentation (EDF) for Comminution of Steel Casting Ladle’s Refractory (MgO-C) and Cement Rotary Kiln’s Refractory (Hercynite)” gained the attention of four students, who decided to validate this technology for the comminution of refractories. This group consisted of students from China, Chile, Pakistan and Finland. – a diverse group that brought different fields or expertise, different cultural backgrounds and different perspectives and perceptions together.
After two months of laboratory work and one month for the evaluation and visualization of the results, the project study was finished. The result is that high voltage pulse power fragmentation is a novel route for the comminution to weaken or fully fragment rocks.
At the PROMISE Summer School in June 2023 we presented the results of the ReSoURCE project study. The audience consisted of PROMISE students as well as professors and scientific staff of all the involved universities in the master programme. Our high voltage pulse power fragmentation contribution knocked the socks off the audience – Although the liberation of the comminuted output fractions has to be analyzed in the next step to evaluate the efficiency of the process.
Myself and my colleagues at the Chair of Mineral Processing are looking forward for getting the next PROMISE cohort in the next summer semester. We are glad that one of the students within the ReSoURCE project study decided to come back to Leoben for writing her master thesis in ReSoURCE.
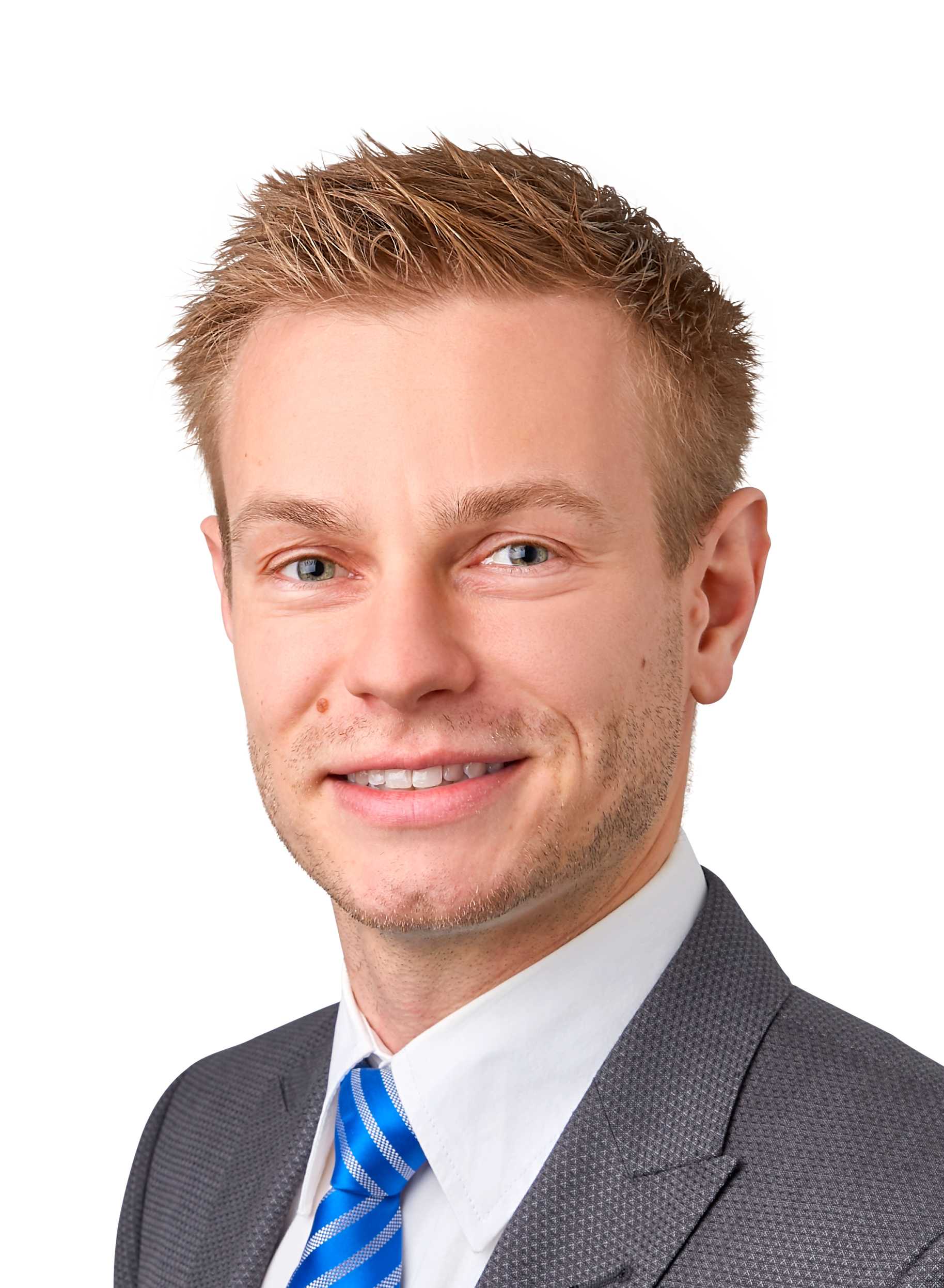
Author’s Portrait
Karl Friedrich
DI Karl Friedrich is research associate at the Chair of Mineral Processing in the area recycling at the Montanuniversitaet Leoben.
Before that he was employed as research associate at the Chair of Waste Processing Technology and Waste Management (area: sensor-based sorting) and the Chair of Economic and Business Management for sustainability management and digitalization.
Since 2018 he participates in the Montanuniversitaet’s PhD Program. The title of his thesis is “Increasing efficiency in sensor-based sorting processes for polymer waste”.
From 2011 to 2017 Karl studied in the Bachelor and Master program “Energy and Environmental Management” at the University of Applied Sciences Burgenland. His elective subjects were “Environmental process engineering” and “Energy engineering and energy economics” and his master thesis belongs to recycling opportunities for glass residues after thermal waste treatment.
Karl’s research interests are Sensor-based Sorting, Mineral Processing, Recycling, Digitalization, Waste Processing, Waste Management, Life Cycle Assessment and Material Flow Analysis.
The year 2023 turned out to be a fantastic year both personally and professionally. In January 2023, we stepped into a new year after successful completion of half project year in 2022. In 2023, we have submitted a total of 9 deliverables and achieved 2 milestones; indeed, it was once again a great journey to work with our consortium members.
Since we had our first deliverable for the year 2023 by end of February, RHI Magnesita’s ReSoURCE team was very busy right from the start of the year in summing up the results for the deliverable. In March 2023, we had a deliverable in communications and it came out very good. In May 2023, we had two deliverables one regarding technical topics and another one from the communication side, both were successfully submitted in May 2023. In September 2023, we had a little delay in handing in one of our deliverables. It was the first time ever that we had a delay in submission since we could not acquire the required number of survey results for the deliverable. Anyhow, we have informed the project officer in advance and the deliverable will be submitted in November together with other 4 deliverables.
As a project coordinator, I was quite busy from Mid of April with arranging the M12 meeting for the consortium by sending emails and coordinating the work package meetings in Porsgrunn. The M12 meeting in Porsgrunn took place from June 12 to 14, 2023. It was very well organized by our consortium partner SINTEF. The whole consortium had great technical discussions and it was wonderful to meet and greet the consortium personally.
On 10th October 2023, we got the official invitation from European commission for the first review meeting in February. Our EU project officer was very kind to accept our choice of location to be Aachen since it is a good location to show the progress of the project at this specific period from our consortium members LSA GmbH and Fraunhofer.
We have started our preparations for the first review meeting from mid of October as we are obliged to report both technically and financially. I have arranged several project managements, deliverable, work package and financial update meetings to check if everything is in right place from October until December 2023. I am confident that we will have effectively prepared and summed up the reports by end of December, so it will be ready to be transferred to the European commission long before January 31, 2023. On November 30th, 2023, we have deadlines for 5 deliverables and each of our consortium partner worked hard to get it done. I’m very happy with the way we progressed in the first 18 months.
As the technical updates goes on, I too have some personal changes update in the team. Carmen Loew, our Communications specialist for ReSoURCE was leaving the company at the end of October 2023 and I am expecting my second baby in February 2024. So, we had to search candidates for both positions in October 2023. It was lot of work, but I really enjoyed the smooth transfer that we have planned in both positions to make the candidates comfortable to take on their new jobs. I congratulate both persons for their new jobs and I hope they will have a great time working in the project ReSoURCE. I will be away for my maternity leave from Dec 16th, 2023, until end of Dec 2024 and will be back to the project ReSoURCE in January 2025. Earlier, I was eagerly waiting for the first review meeting in February 2024; anyhow, God has other plans for me as now I am excitedly waiting for my second baby to arrive in February 2024. I wish you all and especially my consortium members warm and cozy holidays with showers of snow and happiness blooming around the homes 😊.
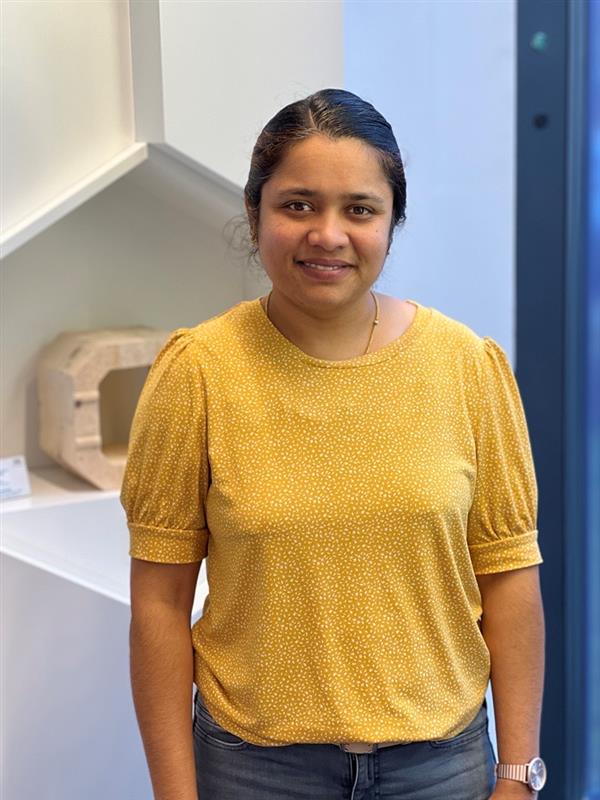
Author’s Portrait
Saranya Azhaarudeen
Dr. Saranya Azhaarudeen studied at the Technical University of Denmark and has a PhD in Surface and Coatings Technology. She is Innovation Management Professional at RHI Magnesita and Coordinator for the project ReSoURCE.
ReSoURCE’s main objective is to increase the recycling rate of refractory bricks. Broken-out refractories already have a non-negligible fines content when they are first removed, for example from a steel casting ladle. In further processing, RHI Magnesita crushes them into smaller blocks with a maximum edge length of 120mm, to be sortable by the new equipment. These single grains are sorted with the so-called Demonstrator A, which is also developed and evaluated during the project. The crushed bricks are then screened to minimize dust generation and contamination during the sorting process. In each individual step, fine particles are thus created. During the ReSoURCE project, we speak of fines at grain sizes smaller than 5mm.
Demonstrator B (Demo B), which we consider in this post, is designed to process these fines, and contribute to an enrichment of the desired material classes. Demo B can be seen as a scientific approach to enrichment rather than an industrial facility. Its purpose is to analyze and evaluate the handling and sorting of fine parts in a sorting plant. All modules of the plant are designed in such a way that they can be interchanged in their sequence. This allows the material flow to be tested in different configurations of the plant, to find the optimal setup for a later industrial approach.
In the sketch a first preliminary design of the setup is shown: The fines that are generated during the above-mentioned process steps are collected in a hopper (1). With the help of a pump (2) an air flow is generated which transports the fines through the system. During this process, the fine parts pass-through various stations.
First, the chemical composition of the different grains will be examined as a bulk by a measuring system (3) using Laser-induced breakdown spectroscopy (LIBS) provided by LSA. With LIBS, the chemical composition of a material can be analyzed in a contact-free and non-destructive manner. After analyzing the composition, the sorting of the fines can start. The sorting can be distinguished in two different types of sorting methods, direct sorting (4) and mechanical sorting measurement (5). Direct sorting in this application means, a sorting based on physical or chemical parameters and requires no additional sensor in advance.
Examples for the direct sorting modules could be a vibrating table classifier, that separates smaller from bigger grains by feed them through holes with different sizes. Mechanical sorting method could be implemented by using a mechanical flap, that divides the fines-flow into two different collecting bins, corresponding to the chemical composition. The sorting methods are developed and tested by SINTEF. If you want to learn more about SINTEF’s work, check the blogpost Powder Classification.
The sorted material is finally conveyed into collecting bins and can be processed further by RHI Magnesita.
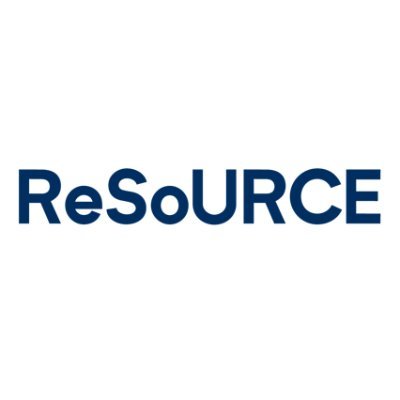
Author’s Portrait
Carsten Coenen
Carsten Coenen is a development engineer at LSA – Laser Analytical Systems & Automation GmbH (Germany). He is working on the mechanical design and the implementation of high-precision 3D-printed parts for use in optical modules. Since 2022 he has been working at LSA. Previously, he completed an apprenticeship as an electronics technician at RWTH Aachen University and received his B.Eng. Mechatronics and M.Sc. Mechatronics from FH Aachen University of Applied Sciences.
During his studies, he already worked as a working student at RWTH Aachen University and at AGVR GmbH (Germany). Since he joined LSA, he has also been involved in EU projects (e.g. REVaMP and ReSoURCE).
Julio Hernandez and Dennis Adamek from Norsk Elektro Optikk, Oslo (NO) came to visit RHI Magnesitas’ researchers in Leoben (AT) to help install a complete hyperspectral imaging setup and train the colleagues in Leoben in how to obtain high quality hyperspectral imagery.
As you might recall from earlier blogposts (see for example the blogpost from Friederike Koerting: Our Samples in the Light of Science), hyperspectral imaging is a technique that can detect spectral information with high resolution (typically several hundreds of narrow, contiguous spectral bands) for each pixel in an image, allowing for the identification of specific materials or substances based on their unique spectral signatures. Hyperspectral images can reveal intricate details about the composition and characteristics of the imaged objects or scenes.
The system that was installed in Leoben carries both the HySpex VNIR-1800 and the SWIR-384 cameras, which, when combined, allow to record spectral information from 400-2500nm, covering the visible, near infrared and short-wave infrared spectral regions. Samples are illuminated by two strong halogen lamps that provide light across the complete spectral range. The spectral distribution of the light reflected from the samples depends on the chemical characteristics of the material. As such, it contains information on the composition of the samples and can be used to identify specific properties. The cameras used for collecting this reflected signal are line-scanner imaging spectrometers capable of orderly diffracting a broadband input light signal and map it onto a digital sensor. Spatial and spectral information is acquired simultaneously from a linear section of the imaging area, and either movement of the camera with respect to the samples or vice versa provides the second spatial dimension for forming a 2D image with spectral information stored in the third dimension of a 3D digital array.
With this lab setup the researchers at RHI Magnesita will be able to scan lots of samples during the next months and collect large amounts of data on the different kinds of refractory materials investigated in this project. On a controlled data set also reference measurements like XRF or Micro-XRF will be performed that will serve as a ground truth to validate the hyperspectral data. Subsequently we will use all these measurements to train classification and quantification models that will be able to deliver information like brick type, composition or potential contamination of previously unknown samples, enabling automation of the refractory recycling process the ReSoURCE project is aiming at.
During the two days of this visit the team had fruitful discussions about refractory material characteristics, hyperspectral imaging best practices and strategies to distinguish certain refractory material types based on material-specific spectral features. Time allowed to start scanning and investigating selected used refractory brick material samples from both cement rotary kilns and steel casting ladles. The samples were prepared in form of brick slices and irregular breakout material chunks to account for different sample geometries. The high carbon content and thus dark color of the steel casting ladle bricks were challenging to scan this time as only a white reference panel was available for testing, which quickly saturates at camera integration times that are necessary to obtain enough reflected light from these samples. Reference panels are used to calibrate the hyperspectral images to true reflectance to provide absolute measurements and inter-comparability between different hyperspectral systems or other spectroscopic instruments. Within the next week the RHI Magnesita team will get suitable darker reference panels as well to account for various brightness of the investigated refractory samples. The higher brightness of the cement rotary kiln brick samples, however, made it easy to obtain high quality images. An interesting feature of these kinds of bricks is the type of spinel included in the brick matrix. The spinel material is not susceptible to infiltration and contamination during the burning processes, so an interesting experiment will be to see if the spinel type can be used as a marker in the recycling decision process.
We are looking forward to further discussions as soon as more data is available and hope that the experiments performed by the colleagues at RHI Magnesita will clarify brick sorting criteria and help build reliable and accurate models to improve refractory material recycling and reach the goals of the ReSoURCE project.
Also, our project partners from Montan-University Leoben (AT) are invited to take part in the experiments and get hands-on experience with the system now installed in Leoben.
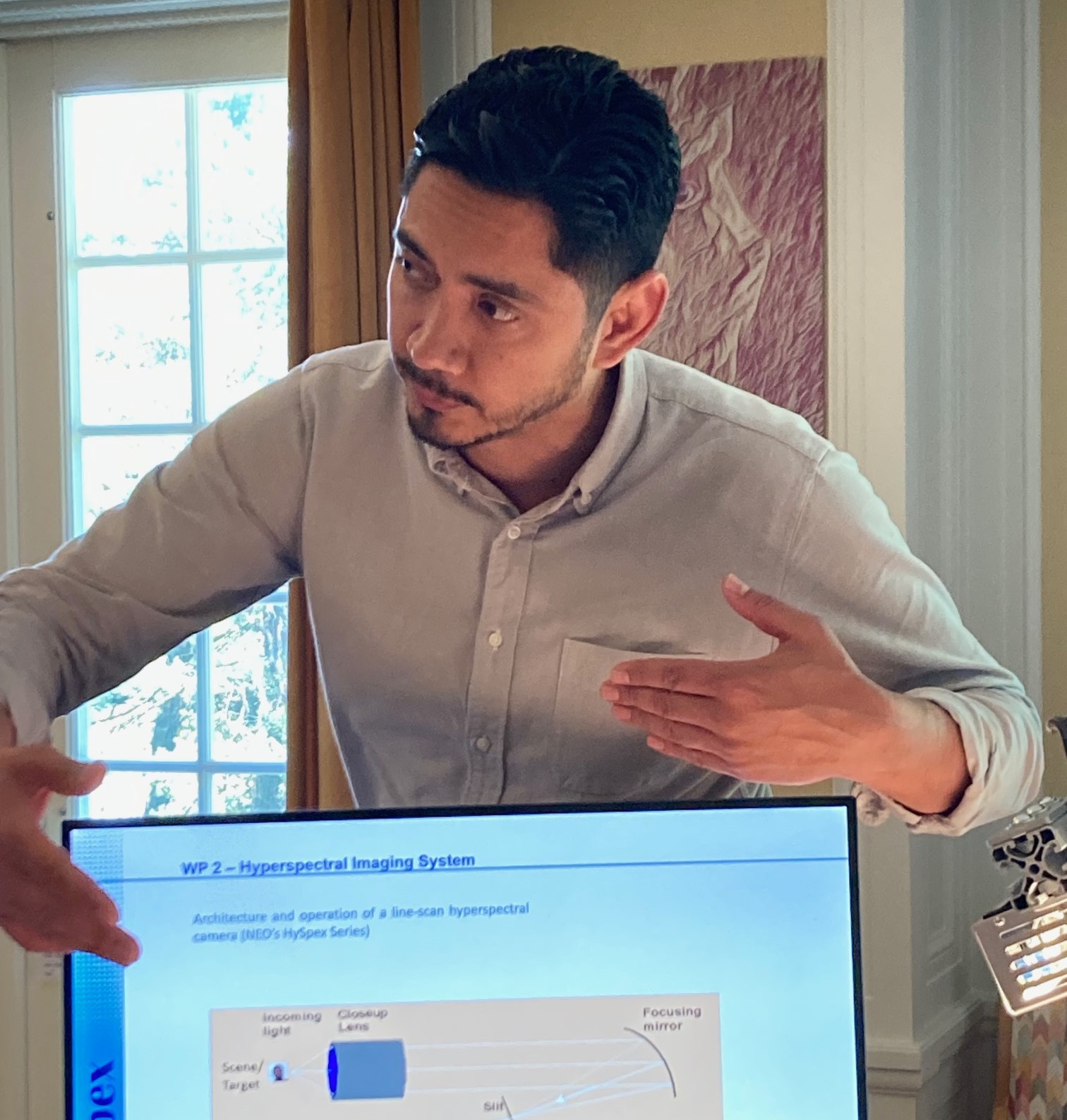
Author’s Portrait
Julio Hernandez
Julio Hernandez, MSc, is a senior research scientist at Norsk Elektro Optikk AS with over 15 years of experience in the field of hyperspectral imaging. Julio has worked developing scientific-grade hyperspectral cameras and data acquisition systems for a variety of applications within remote sensing, defense, industry and biomedical research. He is currently Manager of the Hyperspectral Applications department at HySpex, focused on developing customized solutions for end-users and promoting the adoption of hyperspectral technologies in new markets. Julio studied Physics at the Autonomous National University of Mexico (Mexico) and Nanotechnology at Chalmers University of Technology (Sweden) with specialization in quantum information systems.
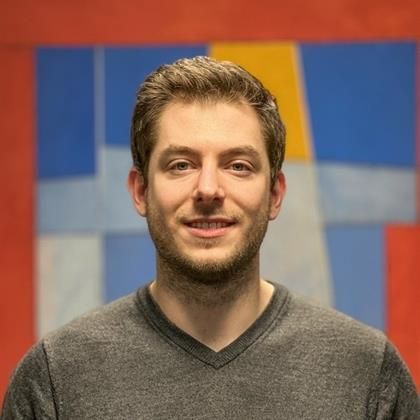
Author’s Portrait
Dennis Adamek
Dennis Adamek, MSc, is an application specialist at HySpex/Norsk Elektro Optikk AS, focusing on enabling hyperspectral imaging technology for industrial applications. Within the ReSoURCE project he is supporting experiment design, hyperspectral system installations and hyperspectral data analysis. He holds a master’s degree in physics from Friedrich-Schiller University Jena, Germany, with a specialization in optics, photonics and spectroscopic data analysis.
Ahead of Saranya’s maternity leave starting in December 2023, our former science communicator Carmen Loew (CL) did an interview with Ramona Oros (RO), the interim project manager for ReSoURCE at RHI Magnesita.
CL: Hello Ramona, welcome to the ReSoURCE Team! Did you have the chance to meet some of the new colleagues?
RO: Thank you, yes, I met Alexander and am looking forward to meet more people.
CL: Ramona, what is your professional background? Are you a material scientist?
RO: Yes, I am a telecommunication and material scientist. I have a PhD in Telecommunication and Material Sciences. I also have master’s degree in international business administration.
CL: I assume that this is not the first time that you are working for an EU project, correct?
RO: Yes, that is correct. I have worked with EU funded projects for the last 12 years. I have been involved with around 20 EU projects and fifteen different funding schemes addressing research and innovation topics covering education to industrial relevant topics.
CL: Are you familiar with sustainability topics?
RO: Yes, I worked at EIT Digital before. EIT Digital’s (https://www.eitdigital.eu/our-community/purpose/) goal is to create a strong digital Europe that upholds European values of inclusivity, fairness, and sustainability. For this, the focus was on building the next generation of digital ventures, products, and services, while also nurturing digital entrepreneurial talent to address the digital transformation challenges and green transition at the forefront of digital innovation.
CL: What do you find most interesting about ReSoURCE?
RO: I know that the availability of raw materials is a concern in the EU, and what ReSoURCE is aiming addresses this concern while considering to reduce CO2 emissions and energy consumptions.
CL: Our project is an international one. Your name suggests that you also have an international background. Correct? If so, where are your roots and in which countries did you live before?
RO: Indeed, I’m Romanian by origins and moved abroad after finishing my education. I’ve started my career in Austria from where I moved to Belgium to come back to Austria 5 years later. I can say that Austria is for me home, and where I have the slopes close as I love skiing. During all my journey I’m worked physically and remote in international team across Europe and not only.
CL: What are you most looking forward to?
RO: I’m excited to be part of this team and bring my contribution to such a highly innovative project. I’m looking forward to meeting the project colleagues and start a fruitful collaboration.
CL: Thank you for taking the time to answer our questions. I wish you all the best in your new role.
One might think that last year we took and analyzed more than enough samples to have sufficient material to train the sensors for the automated sorting system. But an essential piece of the puzzle was still missing: a certain type of refractory brick that RHI Magnesita installs in steel casting ladles. So far we have only been able to sample used MgO-C bricks (as Stefan Heid explained in his blog post Appearances are Deceptive), but not used alumina-magnesia-carbon bricks (AMC, i.e. alumina-based bricks with carbon bonding). However, separating these types is extremely important, as incorrect throws and thus contamination in our recycled secondary raw materials lead to problems in the production of new bricks.
Since AMC bricks are rarely delivered to our MIRECO location in Mitterdorf, we went to a MIRECO site in Bremen for our fourth sampling campaign. There, my colleague Florian Feucht (Montanuniversität Leoben) and I were not able to visit the beautiful Hanseatic city of Bremen, but we were able to plan the sampling of almost 6 tons of material and gain very interesting insights into on-site recycling. Unlike in the recycling center, where different breakout materials from different customers (steel and cement industries) are sorted and processed, in on-site recycling only the material from one customer is sorted. In Bremen, the sorting was done directly at the steelworks and the majority of the material was reused for various products, e.g. metallurgical additives. This is also a clear difference, as the material from the recycling center is largely used in our own refractory products. Like in the recycling center, some of the breakout material must also go to landfill. This can be for various reasons, e.g. material that is too fine or cannot be sorted sufficiently.
We also had to approach sampling in Bremen a little differently than in Mitterdorf. Since there were only two of us on site this time, manual sampling of a 150 t pile was not realistic without severely disrupting ongoing operations. It was therefore agreed that this time the sampling would be carried out directly from the conveyor belt of the sorting system. Adjusted to the full amount of breakout material, a total of 6 big bags were filled with unsorted bricks at regular intervals. This ensured that samples from all different parts of the pile were shipped to us in Austria. We are very excited to see what valuable insights we can gain from this sampling for automated sorting.
Another interesting aspect of our trip was an impromptu crash course in hand sorting MgO-C and AMC stones. A visual comparison of the two types makes it clear where the challenges lie in hand sorting. In short, to us, at first glance, everything in the pile looked the same. Nevertheless, in Bremen we were shown how even these two difficult-to-distinguish varieties can be separated manually with a lot of experience. Here it is above all the details that make the difference. For example, AMC bricks are more compact and therefore usually less broken after breaking out of the ladle than MgO-C bricks; The original format (i.e. the original size of the stones) is usually easier to recognize than with MgO-C stones. AMC stones are also harder to break and sound different when struck with a hammer. However, despite our crash course and the efforts on site to introduce us to the depths of hand sorting, we will play it safe and continue to rely on chemical analyzes for our further experiments. In any case, this visit showed us once again how much experience is necessary for solid hand sorting and how complex the recycling of refractory bricks is.
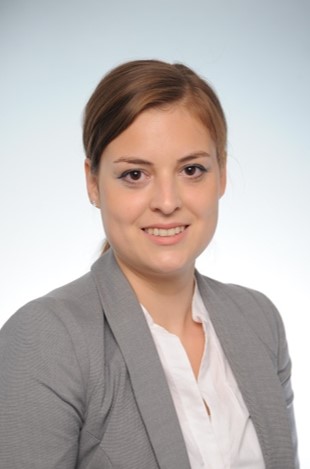
Author’s Portrait
Simone Neuhold
Dr. Simone Neuhold currently works for RHI Magnesita. Before she joined the company she was hired at Pilkington Deutschland AG/NSG Group. Simone studied at the TU Graz Chemistry and Advanced Materials Science, and at the Montanuniveristaet Leoben Waste Management and Waste Processing Technologies. Her research interests are recycling of mineral wastes, materials science and oekodesign.
When a furnace, ladle, or kiln lining reaches the end of its service life, the entire lining is extracted with the aid of heavy machinery, necessitating a robust and somewhat aggressive handling process. This break-out procedure results in generation of fine powders, in which bricks and other refractory products are crushed and mingled with impurities from both the service product and its surroundings. Today, most of this fine fraction typically ends up in landfills.
In our ReSoURCE project, we are actively working on the development of a direct sorting method to maximize the recovery of this material for recycling purposes, primarily as refractory material or for further “downcycling” where recycling is infeasible.
The leftover material consists of particles smaller than 5 mm, with the smallest particle sizes qualifying as dust, which poses considerable challenges in terms of material handling. While there exist several techniques for separating particles based on their size and density, it is critical that the chosen method aligns with the composition of the raw material and the desired product fractions. The refractory leftover material presents a complex blend of particles encompassing a wide range of sizes, refractory products, and impurities. Thus, experimental testing to ascertain the efficacy of the method and to optimize the separation process is crucial.
At SINTEF, we are well-equipped to conduct comprehensive testing on the separation, transportation, and handling of powders, from the lab-scale even up to the pilot scale. Initial characterizations of the leftover materials have already shown good fluidization properties within the smallest fractions, measuring less than 1 mm. Experiments using the multi-chamber fluidization classifier (a test rig developed by SINTEF Tel-Tek, former Tel-Tek) has been used to explore the potential for utilizing this technique both for dedusting and as a purification step. The image below illustrates how dust and lighter particles are carried upward within the air stream, while the coarser and heavier particles are transported horizontally and collected separately.
To further enhance the efficacy of the separation process, SINTEF with help from consortium partners have conducted detailed analyses of the particle size distribution and chemical composition of both the fine and coarse fractions. Evaluating the analysis will help us optimizing the separation process towards a cleaner leftover fraction where recycling can be possible.
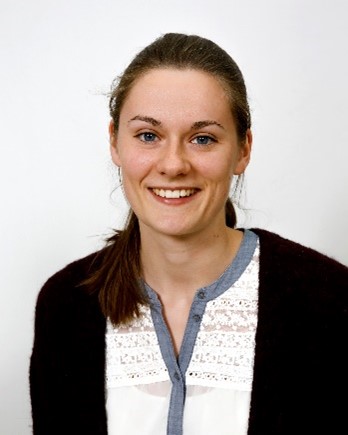
Author’s Portrait
Kristin Søiland
Kristin Søiland is a research scientist at SINTEF Industry in Norway. She has a master’s degree in chemical engineering from the Norwegian University of Science and Technology, specialised in analytical chemistry. At SINTEF her main research areas are within circular economy and powder technology. Prior joining SINTEF she worked in a paint company developing more environmentally friendly coatings.
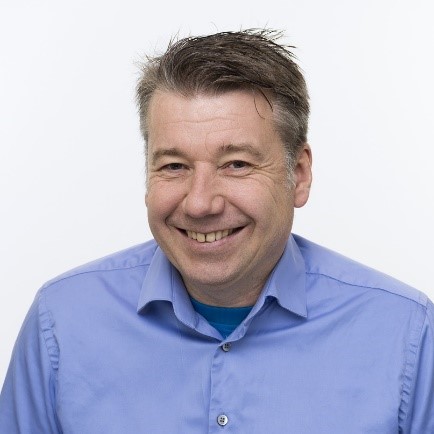
Author’s Portrait
Franz Otto von Hafenbrädl
Franz Otto von Hafenbrädl is a research scientist at SINTEF Industry in Norway. He has several years of experience in powder technology and powder characterization. He started to work with powders in 1996 in Tel-Tek, that is now part of SINTEF. He has a master’s in science of process engineering and a bachelor’s in chemistry from University of South-Eastern Norway.
In a previous Blogpost “Survey on waste Refractories – Tell me about your data”, we talked about the difficulties of acquiring suitable data for our material flow analysis (MFA) with which we want to describe the European waste management system.
Briefly summarised, this type of data is hard to get. This is why we created and promoted a survey to query information of interest. But since response rates of surveys are such a thing, we had to came up with more ideas on how to get data for our MFA. To give a little more context, response rates are often very low, sometimes too low to be statistically significant, but they can always give a glimpse of a possible trend and help to get a first insight. Especially when we look at the final fate distribution, i.e. the distribution of spent refractories among the categories recycle, reuse and landfill as data regarding this topic is rather rare. But we did not want to rely solely on survey data. That’s why we created a multi referentially or three-pillar-approach as we call it, since this serves as a foundation for our MFA. One of the pillars, to be more precisely the third pillar, is of course our survey results. If you have not done so, please participate in the survey. We’d appreciate your support.
The first pillar is based on the limited literature we found regarding this topic and the second pillar is an assumption based on production data. The first pillar relies on peer-reviewed research reports, books and partly on grey literature (conference papers or presentations). As a brief explanation, grey literature lacks a form of quality control like the reviewing process which is mandatory when publishing in renowned journals, therefore it is important to carefully evaluate the reliability and credibility of grey literature sources before using them in research.
For the second pillar we included even more grey literature like annual company or association reports of the steel, cement and refractory industry. We then used the reported production data and the reported specific consumption to calculate the annual refractory demand. Every information that was not reported in annual reports was taken from the first pillar.
For every pillar we created an individual MFA describing the differences and rating the confidentiality of the data and how likely it is that a certain MFA represents reality.
Some may wonder why we go to such lengths to obtain data when there are companies that conduct market research and possess the data that we need. Well, this companies sell this data for a fortune which subsequently may compromise the transparency, future comparability, and repeatability as not every research institute is able or willing to pay such high amounts of money. However, it’s hard to find ways to improve and create effective waste reduction and recycling strategies without accurate and comprehensive data.
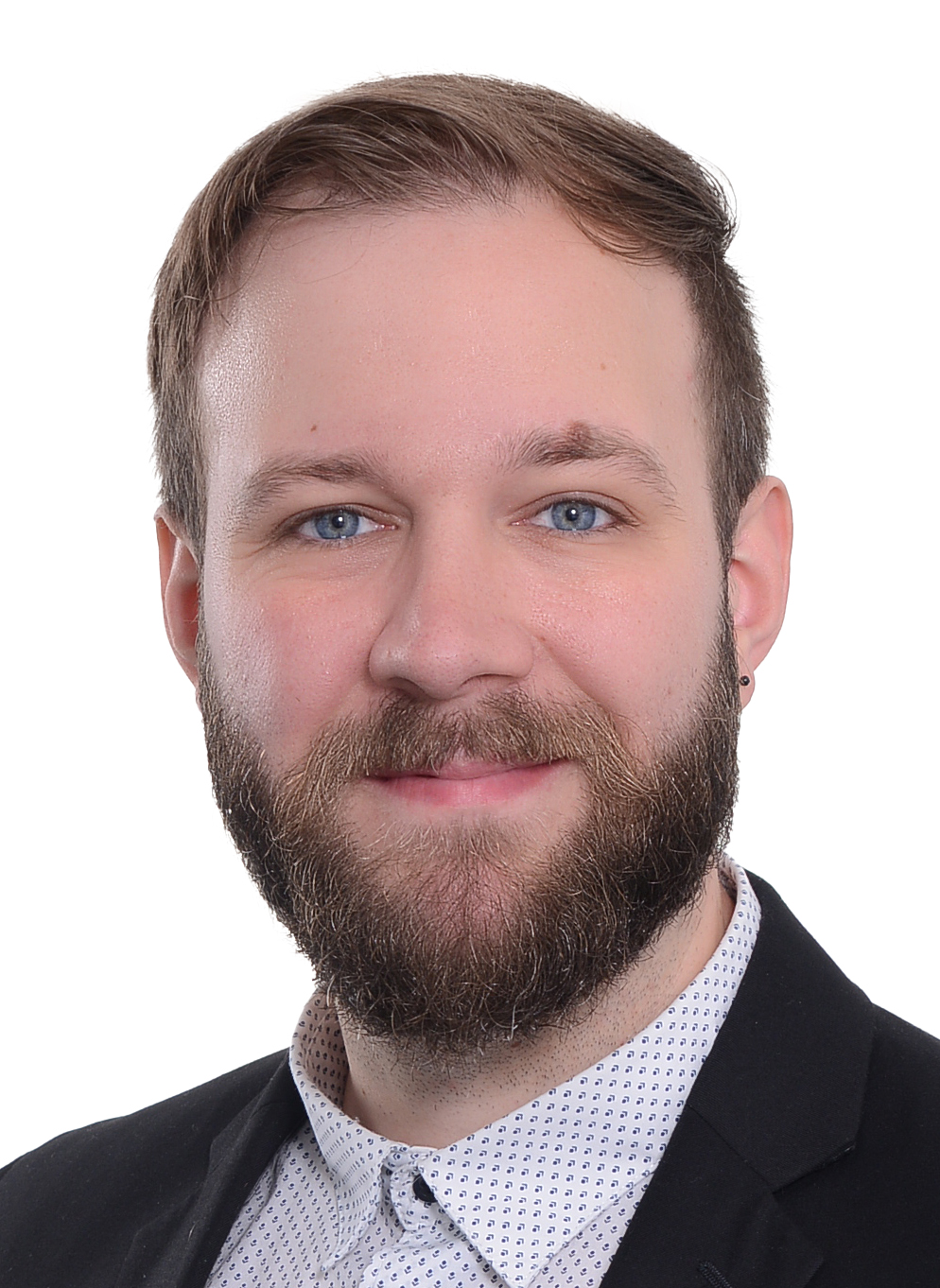
Florian Feucht
DI Florian Feucht, is research associate at the Chair of Waste Management and Waste Treatment at the Montanuniversitaet Leoben, and part of the Workgroup: “Environmental remediation and mineral waste”. Since 2023 he participates in the Montanuniversitaet’s PhD Program. From 2018 to 2023 he studied “applied geoscience” at the Montanuniversitaet Leoben and wrote his master thesis about “Chemical-Mineralogical Characterization of Ladle Slag from voestalpine Linz”. From 2014 to 2018 he studied at the University of Vienna Earth Sciences and wrote a bachelor thesis on the subject: “Petrological and Petrographical Ínvestigation of Mafic-, Ultramafic Rocks from the Dunkelsteinerwald, Gföhler Unit, Moldanubic” Florian’s research interests are chemical-mineralogical characterisation of mineral wastes, Mineralogy, Slag mineralogy, Recycling and Waste Management.
It might not be obvious why a refractory recycling research project is presented at an international conference in Belfast that is mainly focusing on a cultural history context. But to compare science communication and outreach in an industrial research project to projects in humanities, it was the perfect place to be.
Before I joined the ReSoURCE team as a professional science communicator, I worked more than 20 years in archaeology – a discipline that is traditionally keen on following an interdisciplinary approach and that was in the projects I was involved in almost always linked to Geology, Physics, and Biology to name only a few. In former projects I had seen that there is sometimes a difference in communication and outreach but also in general management tasks in the different entities. In the project ReSoURCE we have partners from very diverse backgrounds; there is a university, several private research institutes and smaller businesses of different sizes and the large refractory company RHI Magnesita. All of them have their own understanding regarding the need of science communication and they also have very different set-ups when it comes to professional communicators in their entity. To take a closer look at the similarities and differences not just between our partner entities but also in general between humanities and industry, I handed in a paper at the EAA session “Coordination, Dissemination, and Communication” by Helena Seidl da Fonseca at the EAA in Belfast this year that got accepted.
I must say that this was the most interesting topic I have worked on in a while. For example, coming from entities that are most closely connected to universities and their academic research, I was not aware of the large investment businesses like RHI Magnesita make in research and development.
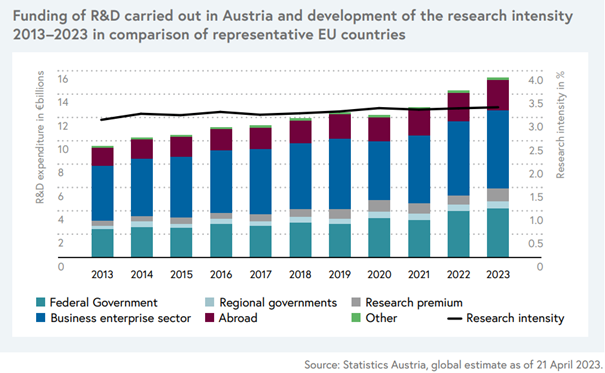
According to Statistics Austria, the business enterprise sector invests – traditionally – more than the federal and regional governments together. Even taking the research premium into account, the businesses are still in the lead. But why do they invest so much money? The main reasons for industrial entities to invest in research are innovation and competitive advantage, product development of course, cost reduction, to gain protected intellectual property, maybe to improve their image and reputation, and surely many more. In humanities, the main reason for an investment is educational value, preservation of cultural heritage, legal and ethical responsibility amongst others. But I realized that one of the reasons here is, too, improving the product and adjusting it to the customer’s needs. This phrase might sound weird, but it is true: the questions we ask in Humanities that are the starting point for our research are usually inspired by the society we live in (our customer), and to which we want to contribute to with our research. The outcome – hopefully – ends up being presented in an exhibition or a documentary or being published in a book (our product).
Much like the reasons for the research itself, the motivation for science communication is similar, too, to a certain degree: reputation of the entity or the participating scientists, leadership in their specific field, and employer branding – all these are true for both. While they also both try to reach and engage the broader public, I assume that this might often be more focused on specific stakeholders when it comes to industrial research. In general, the humanities are much closer to the educational and purely academic sphere than science conducted in the business or industrial sector. Of course, this also influences the nature of science communication in the various areas.
But the main difference that I have seen so far has much less to do with these aspects and more with the resources that are available for scientists. It has a massive impact on the science communication if you can collaborate with a professional communicator and have access to their professional knowledge and tools. A press release for example that needs to be send out is daily business for every university and every bigger company. The communicator will let you know what the best timing is, how such a text needs to be structured, which pictures you can and should use. They will take care of sending it out and it will magically appear on online platforms and in print. This “magic” is quite often done by press service agencies that are well paid for this kind of support. It is a very different game when a small entity, who has no expert on the team with this kind of specific knowledge, must carry out a similar thing.
This is an even bigger problem when we consider that most scientists are not educated in communication. This is unfortunately true no matter the field or specification. All students learn a very special way to communicate when they are trained at the university. It is almost a special language with its own commonly (common only in the specific field) know wordings and sometime even grammar. On top of that, scientists structure their content differently: in the spirit of transparency and with the goal to allow others to gather information first and discuss opinions later, they usually give a lot of background information before they come to the important points. Communicators are well familiar with the pyramidal structuring of communication content, which mentions the core message first and gives more detail only afterwards. Basically, scientists are trained to communicate in exactly the opposite way. One conclusion I made in my presentation is hence the importance to make sure that professional communication is part of the curriculum in all disciplines.
The result of my deeper insight into this topic is that the differences between what looked so different from the outside are actually not that big. The biggest difference is the size of the company, as smaller companies simply don’t have the resources they need to communicate professionally. We must therefore continue to work on training scientists and ensuring that they themselves learn to communicate with the general public.
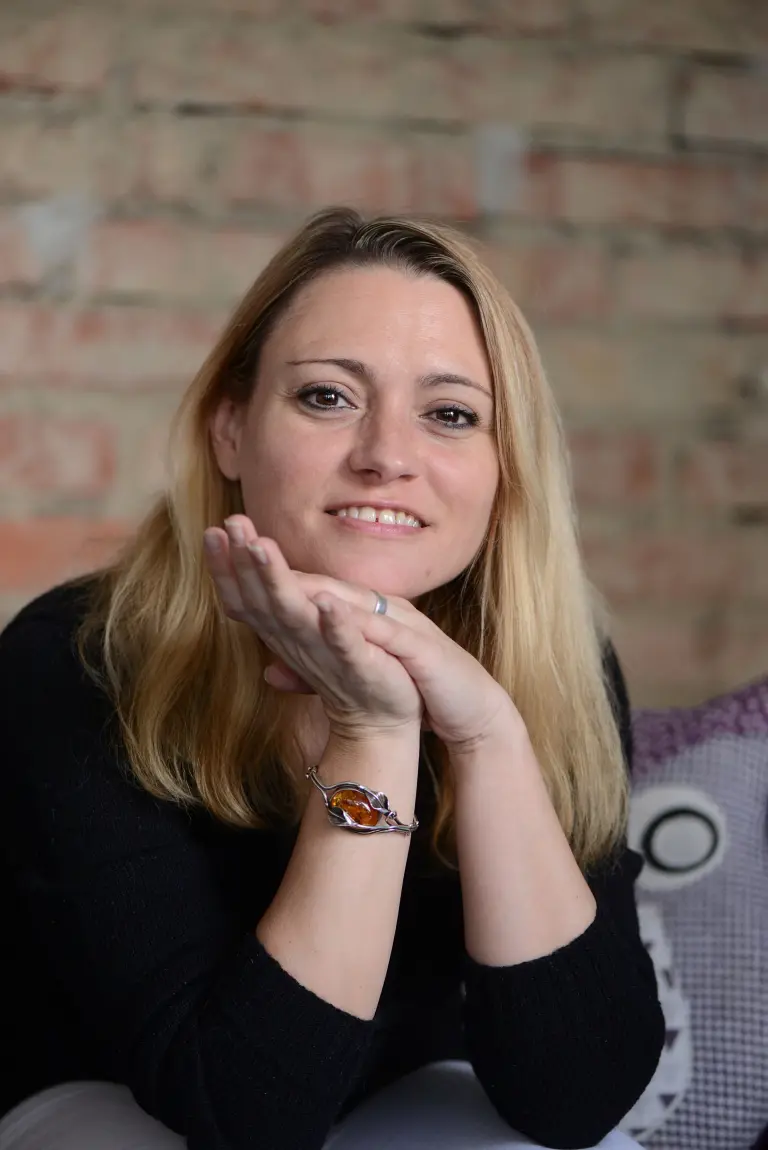
Author’s Portrait
Carmen Loew
Carmen Loew, Magistra Artium, is the project ReSoURCE’s science communicator. She studied Archaeology at the Universities of Saarbrücken and Bamberg and managed projects in research and rescue archaeology in Germany and France before she focused on science communication in 2015. She is a certified PR manager, Fundraising manager, Marketing & Sales assistant, and cultural educator. Her (research) interests are science communication and outreach, crisis communication as well as intercultural communication.
Full of excitement for the first hands-on experience on Laser-induced breakdown spectroscopy (LIBS) measurements, we recently welcomed Joachim, Heinrich, and Volker from LSA at our RHI Magnesita Technology Center in Leoben. With them, two huge wooden boxes arrived, delivered by a shipping company. The boxes contained a LIBS demo system that was set up at our facility and was available to us for three weeks of test measurements. As the boxes were carefully opened, anticipation filled the air. LIBS is a cutting-edge analytical technique that offers unique insights into the elemental composition of materials. The system utilizes focused laser pulses to create a micro plasma on the material’s surface, causing it to emit light. This emitted light is then analyzed with a spectrometer to identify the elemental composition of the sample.
The LSA team gave us a comprehensive training, so we can use these tools professionally to study our refractory material and to create the baseline for a fully automated sorting system. With these measurements the system should be able to recognize target specifications of pursued sorting classes, so we chose to train with a variety of raw materials and brick types used in the steel and cement industry. The outcome of our test runs were a qualitative overview about the response of the LIBS signals to the different sample types. Combining LSA’s expertise in LIBS technology with our in-depth knowledge of refractory materials proved to be the bedrock of our success. Their wealth of experience not only accelerated our understanding of LIBS but also provided valuable insights into optimizing the technique for our specific needs. Together we were determined to unlock the full potential of LIBS and its application in our industry.
Beyond the technical marvel and scientific significance, our practical experience with LIBS measurements has been an exhilarating journey. Watching the laser beam strike the sample like dazzling fireworks, filled us with awe. The sparks emitted during the process fueled our curiosity and passion for material science. Embracing this spectacle of science, we are motivated to explore further, pushing boundaries, and fostering collaborations that illuminate our path ahead. Stay tuned for more information about the progress we make!
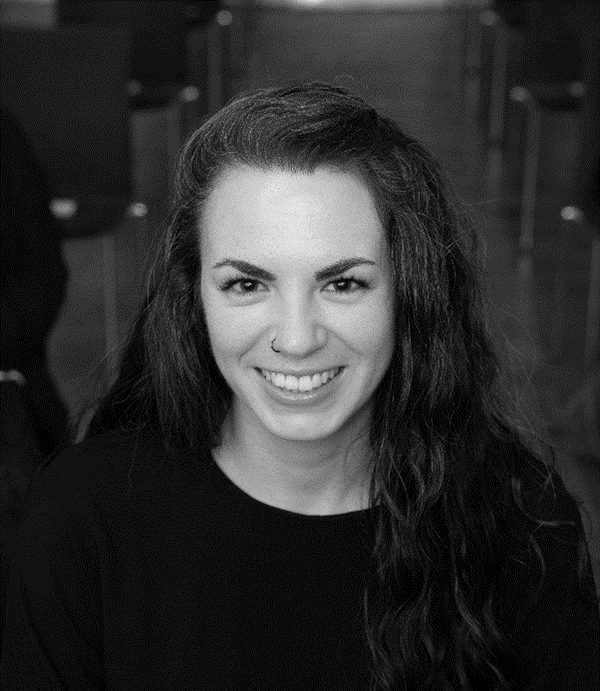
Author’s Portrait
Katja Goetschl
Dr. Katja Goetschl studied Geosciences at the Graz University of Technology, specializing in the field of Experimental Geochemistry and the fundamentals of CaCO3 mineral (trans)formation. She joined RHI Magnesita as Pioneer Research Associate in 01/2023.